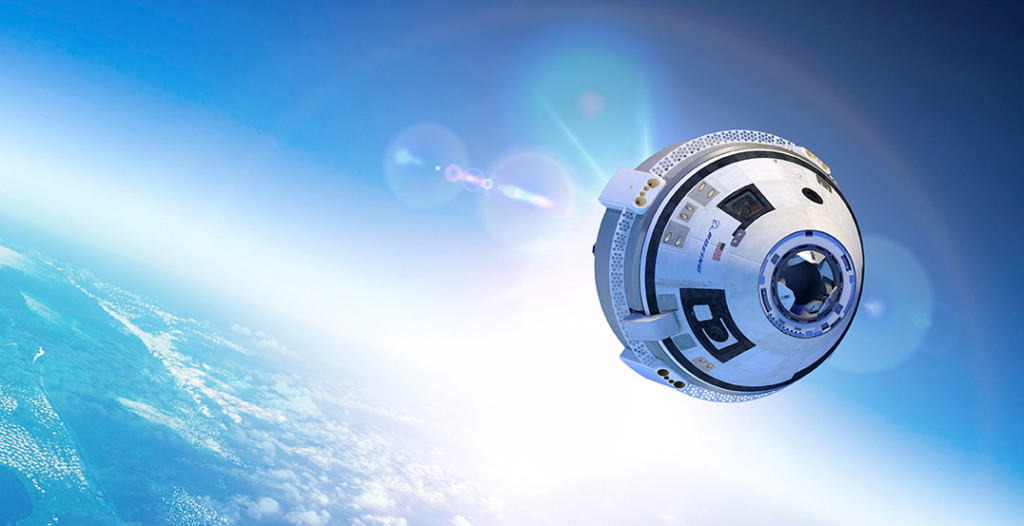
The Boeing CST-100 Starliner crewed vehicle, to be used for LEO destinations. (Boeing)
NASA has identified specific scientific and technical challenges for the journey to Mars through rigorous studies, including an ongoing series of architectural trade analyses, external reviews, assessments of deep-space habitation options with international partners, and high-priority objectives of science decadal studies. NASA and our partners around the world have already solved some of these challenges. The remaining challenges will be systematically addressed over the next two decades by the capabilities demonstrated through science missions, on the ISS, and in the Proving Ground as we move toward Earth Independence.
Transportation
Transportation capabilities are necessary to send humans to space affordably and reliably, provide high-thrust access to staging points in cislunar space, and efficiently and safely transport crew and exploration systems on the longer journey to Mars.
Commercial Cargo and Crew: Advances in transportation capabilities are only possible if NASA can shift to a more efficient mode of operations for current Earth-to-LEO transportation. NASA is partnering with commercial industry to make this shift possible. Through a commercial crew and cargo capability, NASA can rely on a less expensive, flexible commercial market to provide LEO transportation services, freeing up resources for beyond LEO and planetary transportation.
Beyond Low Earth Orbit Propulsion—SLS and Orion: A human-class Mars mission will require unprecedented amounts of mass transported farther than any previous human mission to space. A single Mars mission may require several 20-30 mt payloads delivered to the surface to support the crew as well as an in-space habitat, transportation stages, and supplies for round-trip missions of up to 1,100-days. In addition to mass, payload volume is a challenge. To enable cargo missions, NASA envisions a new 10-meter diameter fairing for the evolved SLS to accommodate unprecedented volumes. Commercial cargo services may be used to supplement the SLS’s core role.
In-Space Power and Propulsion: Power is critical for exploration systems; however, it is particularly important for the transportation architecture. Each human Mars mission will require several cargo launches to pre-emplace infrastructure and supplies. NASA expects to use high-powered SEP systems (150- 200 kW) that can transport cargo in a sustainable cadence. SEP requires 50 percent less propellant than chemical propulsion and uses fewer heavy-lift launches. While SEP takes longer than chemical propulsion to deliver cargo, it gives campaign planners more timeline flexibility by providing trajectory options that are less coupled to the 26-month planetary alignment that drives traditional chemical propulsion architectures.
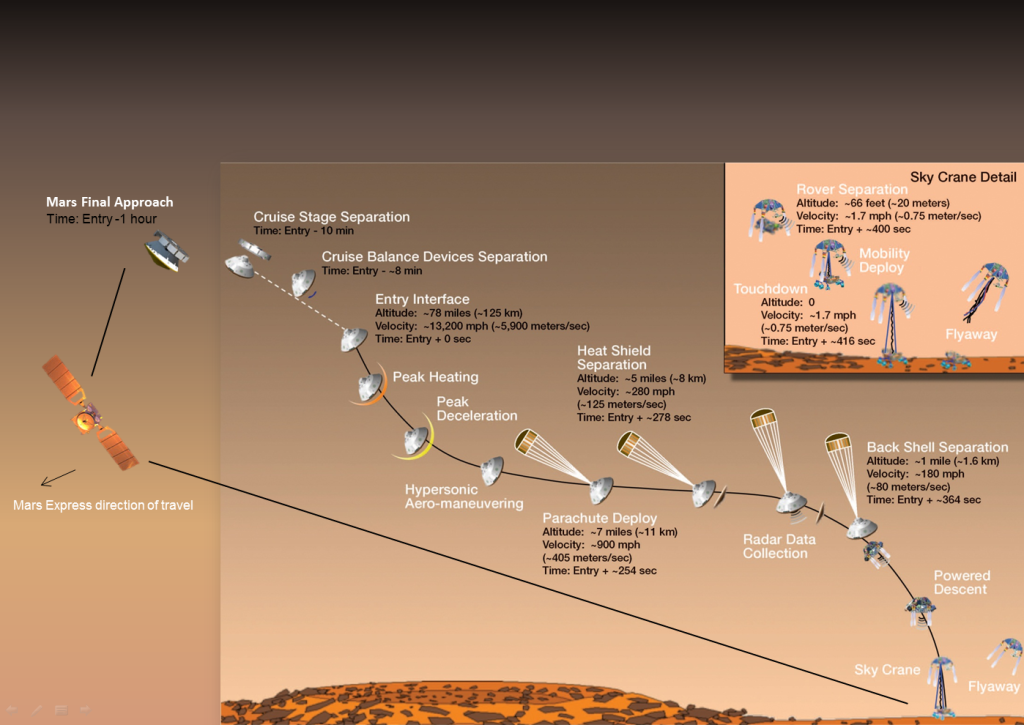
Mars Express support to NASA MSL arrival at Mars.
Entry, Descent, and Landing: EDL is one of our biggest challenges. The revolutionary sky crane landing system used for the Curiosity rover placed just under 1 mt of payload on the surface of Mars. The smallest viable human-scale lander concept is more than an order of magnitude larger, and it may be necessary to land multiple 20-30 mt payloads at a human landing site. Consequently, a completely new approach is needed for human-scale EDL. For instance, supersonic retropropulsion may be necessary to provide safe and accurate atmospheric entry, descent, and precision landing on Mars.
Ascent from Planetary Surfaces: A Mars Ascent Vehicle (MAV) is required to transport crews from the surface to Mars orbit. The MAV drives lander and EDL requirements, which in turn impact in-space propulsion and the total mass launched from Earth, a major driver for mission cost.
Current MAV designs require a minimum lander size of just under 20 mt, assuming propellant can be generated from the Martian atmosphere via ISRU.
The MAV is also critical to crew survival, requiring additional reliability and redundancy, zero boil-off cryogenic storage, and limited maintenance during years of dormancy. Current studies continue to refine our understanding of this critical element.
Communication and Navigation: Currently, Mars robotic rovers have data rates around two million bits per second, using a relay, such as the Mars Reconnaissance Orbiter. The ISS data rate is 300 million bits per second, two orders of magnitude faster. Future human Mars missions may need up to a billion bits per second at 1,000 times greater range than ISS, requiring laser communications to reduce weight and power. In addition, disruption and error-tolerant interplanetary networking and improved navigation capabilities are required to ensure accurate trajectories and precision landing.
Working in Space
Even with 50 years of human operations in space, new capabilities are necessary to sustain productive operations for crew and robotic systems at multiple destinations within the cislunar and Martian environments.
Exploration Extravehicular Activity: New EVA systems must supply basic biological needs during spacewalks, provide protection from hostile environments, and enable comfort, flexibility, and dexterity to support human exploration and investigation of new worlds. These EVA systems will be integrated and tested with vehicle interfaces, such as suitports, using lower-pressure, higher-oxygen atmospheres (e.g., 8.2 pounds per square inch with 34 percent oxygen) for rapid and frequent EVAs with minimum loss of valuable atmospheric gasses. The Asteroid Redirect Crew Mission will be an early opportunity in the Proving Ground to validate new EVA systems when crews conduct spacewalks to collect samples of an asteroid boulder. The potential effects on human health resulting from surface dust and its safe removal will also need to be thoroughly understood.
Human-Robotic and Autonomous Mission Operations: Key features of sustainable pioneering are pre-emplacing equipment, reusing infrastructure, and relying on robotic capabilities to support humans. Robotic systems can help deploy systems, provide assembly, and support maintenance both when crew are present and during periods of dormancy. Robotic systems designed to work with the crew increase productivity, support EVAs, and are critical to crew safety.
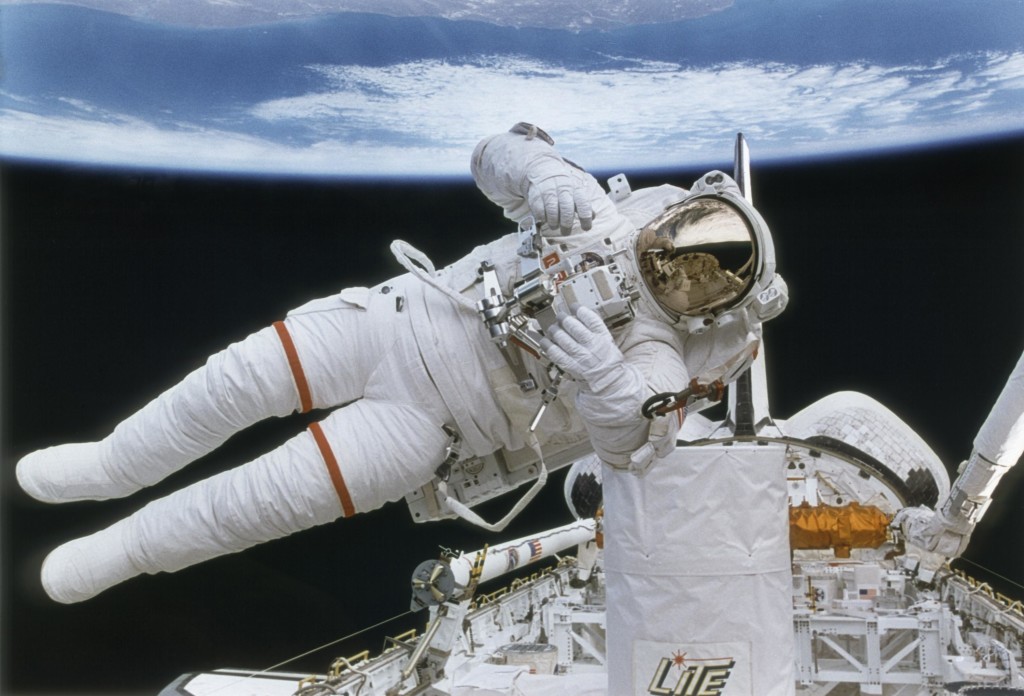
Astronaut Mark Lee tests the new backpack called Simplified Aid for EVA Rescue (SAFER).
In-Situ Resource Utilization and Surface Power: NASA’s science missions have long been searching for water beyond Earth, and they found it: everywhere. Sustainable pioneering must leverage water and other valuable in-space resources to break the logistical chain from Earth. ISRU technology enables the use of local resources, such as water in the form of ice crystals or hydrated minerals on the surface of Mars and carbon dioxide in its atmosphere, to be used as the feedstock for propellant, radiation shielding, and consumables for life support systems. Producing liquid oxygen propellant provides a significant architectural advantage—more than half of a 35 mt MAV mass is due to propellant, which could be produced locally. However, ISRU production for pioneering missions will require significant power to convert resources in an acceptable timeframe. ISRU systems will leverage high-power generation systems, such as solar or fission power, to produce ascent propellant.
Surface Habitat and Mobility: The most important challenge for human pioneering missions is keeping the crew safe for long-duration missions up to 1,100 days. Habitats and associated systems and supplies, including food, clothing, atmospheric gases, and human interfaces, represent a significant portion of any exploration architecture. Habitation includes both in-space transit and Mars surface capabilities. NASA can reduce development costs, increase reliability, and ensure crew safety over a series of missions by reusing and maximizing commonality between the surface, transit, and Mars moons habitats and subsystems.
Staying Healthy
Deep-space crewed missions will not have regular access to the Earth’s resources or the ability to rapidly return to Earth if a system fails. As crewed missions extend farther from Earth for longer periods, the habitation systems must become more reliable for safe, healthy, and sustainable human exploration.
Environmental Control and Life Support Systems (ECLSS): Leveraging the ISS, NASA is focused on demonstrating advanced capabilities for robust and reliable ECLSS, which must operate for up to 1,100 days with minimal spares and consumables. Systems demonstrated on the ISS and Orion will be further validated in the Proving Ground environment and incorporated into a reliable long-duration, deep-space habitation capability.
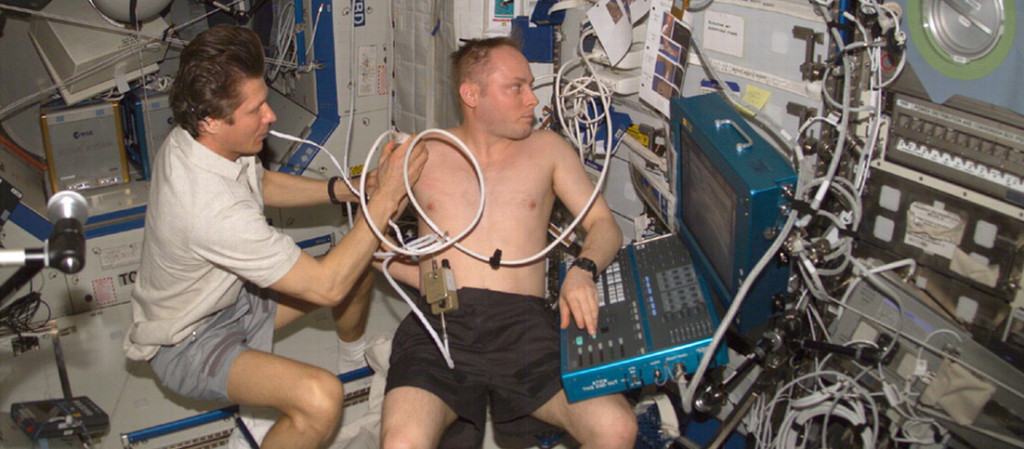
Cosmonaut Gennady Padalka performs an ultrasound exam on astronaut Mike Fincke aboard the ISS
Crew Health: Long-duration human missions, including missions with up to 1,100 days in microgravity, potentially increase the risks of bone loss, atrophy, trauma, neurovestibular issues, loss of clear vision, and illness for the crew. To address these increased risks, crews will require new diagnostic, monitoring, and treatment tools and techniques, including exercise systems and other countermeasures, to maintain crew health. The ISS provides an ideal test bed to develop these capabilities.
Radiation Safety: Outside the Earth’s magnetic field, crew and electronics are exposed to high-energy particles, including infrequent, but potentially deadly, solar particle events and constant exposure to galactic cosmic rays. These high-energy particles can reduce immune response, increase cancer risk, and interfere with electronics. NASA’s Human Research Program is developing methods and technologies to protect, mitigate, and treat the effects of radiation on the crew and their exploration systems. The journey to Mars will be further defined through Proving Ground missions in the next decade, as NASA and our partners retire these challenges and build on the capabilities for sustainable pioneering. We know these challenges are solvable and have a strategy in place for maturing the capabilities to address them as we expand human presence into deep space.