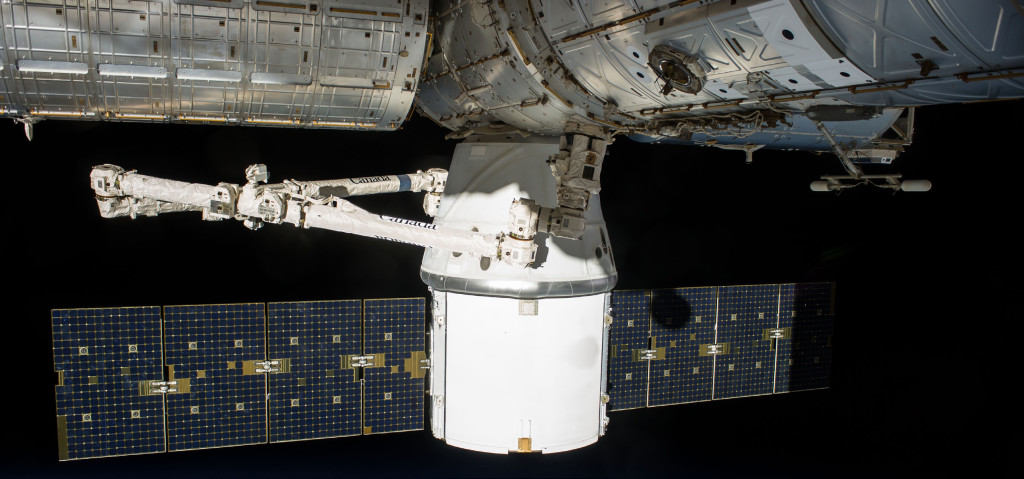
SpaceX’s uncrewed Dragon cargo spacecraft is berthed to the ISS after arriving on September 23, 2014
NASA’s current efforts focus on strategic investments to extend human access to deep space, learn how to operate with reduced logistics, and understand future destinations through science-guided robotic explorers. We will use the Proving Ground to demonstrate capabilities that evolve beyond the Earth Reliant exploration systems currently used on the ISS. As these capabilities are proven, NASA and our partners will further define future missions. Efforts made today and in the next decade will lay the foundation for an Earth Independent, sustained presence in deep space, addressing a challenge worthy of our nation’s expertise, perseverance, and ingenuity.
The following sections describe specific architecture elements in each phase of the journey to Mars: Earth Reliant, Proving Ground, and Earth Independent. These investments, selected with guidance from strategic partners and through the lens of the pioneering principles, enable near-term missions, support resilient space infrastructure, are affordable within NASA’s current budget, and offer multiple opportunities for academic, commercial, and international partnerships. They work together as an interlocking set of capabilities that enable sustainable, affordable, programmatically sound, and technically feasible architecture. Additional detail is provided for the first series of exploration missions, including initial SLS and Orion missions and the Asteroid Redirect Mission. For missions beyond the next decade, insight from ongoing studies is provided.
Earth Reliant
NASA’s current human exploration activities occur in an Earth Reliant frame of operations on the ISS. To begin to break these ties, NASA is leveraging the space station as a test bed to demonstrate key exploration capabilities and operations. Current NASA missions are building on the Earth Reliant capabilities to enable missions for the next decade. The agency is also facilitating a robust commercial crew and cargo transportation capability in LEO, stimulating new markets and fostering an emerging commercial space industry that will mature to support future pioneering missions. As NASA transitions beyond LEO and continues to pioneer space, our vision is that private and public investments will sustain economic activity in LEO and create benefits for Earth through commercial supply and public and private demand.
The First Steps: International Space Station (ISS)
NASA has begun the transition from exploration to pioneering on the ISS. Occupied by an international crew continuously since November 2, 2000, the station has hosted more than 200 people from 17 countries, and is the culmination of one of the largest and most complicated international engineering efforts ever attempted. The ISS is the only microgravity platform for the long-term testing of new life support and crew health systems, advanced habitat modules, and other technologies needed to decrease reliance on Earth. Over the next decade, we will validate many of the capabilities needed to maintain a healthy and productive crew in deep space.
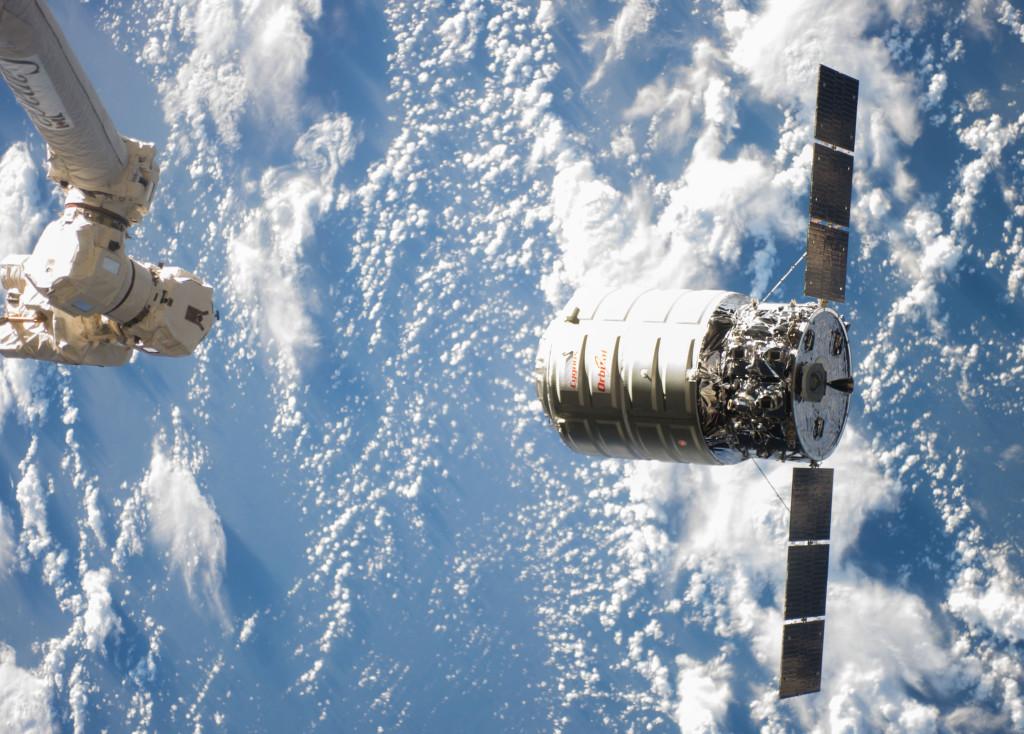
An Orbital ATK Cygnus approaches the ISS during its demonstration flight in September 2013.
Currently manifested or planned experiments and demonstrations include improved long-duration life support for Mars missions, advanced fire safety equipment, next-generation spacesuit technologies, high-data-rate communications, techniques to reduce logistics, large deployable solar arrays, in-space additive manufacturing, advanced exercise and medical equipment, radiation monitoring and shielding, human-robotic operations, and autonomous crew operations. Aboard the ISS, NASA and its partners also conduct targeted research to improve our understanding of how humans adapt and function during long-duration space travel. Current and planned risk-reducing investigations include bone and muscle loss studies, understanding the effects of intracranial pressure changes and fluid shifts, monitoring immune function and cardiovascular health, conducting nutritional studies, and validating exercise protocols.
With these studies, NASA explores the physiology of the human body, preparing for long-duration spaceflight and supporting development of terrestrial drugs and therapeutic practices. NASA and our partners’ activities on the ISS are achieving key milestones and enabling a planned transition to early pioneering missions in cislunar space.
Leveraging U.S. Industry: Commercial Crew and Cargo The guiding principles for pioneering space include leveraging non-NASA capabilities and partnering with industry whenever possible.
NASA’s acquisition strategy for commercial crew and cargo services embodies these principles. The ISS plays a key role as a destination and anchor customer for emerging commercial markets in LEO. Commercial partners, who are maturing their business models and technical approaches by providing critical services for the ISS, will be essential to enabling deep-space NASA missions. The NASA-sponsored Commercial Orbital Transportation Services (COTS) program resulted in the development of new launch vehicles and cargo spacecraft.
Both Space Exploration Technologies (SpaceX) and Orbital ATK have successfully delivered cargo to the ISS using vehicles developed with NASA support. Under the Commercial Resupply Services (CRS) and follow-on contracts, commercial partners are expected to provide about six flights per year to support ISS operations. These flights are win-win arrangements for NASA and industry, as they minimize the need for costly, NASA-unique infrastructure and increase commercial access to space.
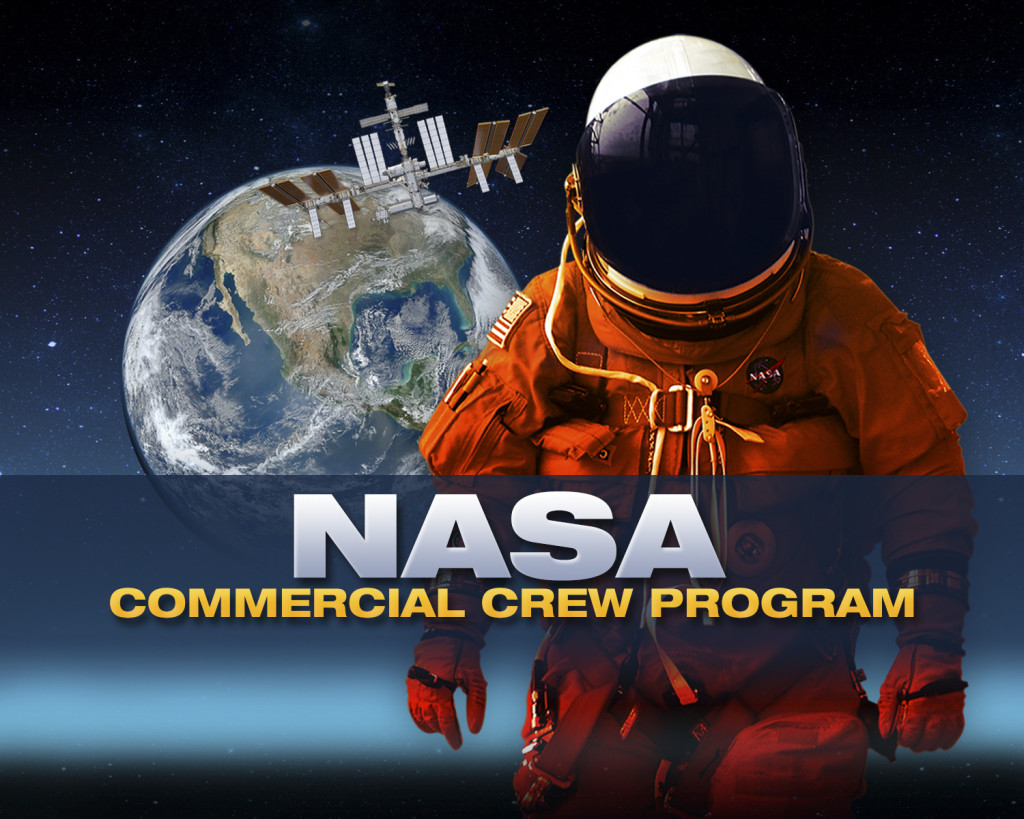
This graphic depicts the goal of NASA’s Commercial Crew Program (CCP)
In September 2014, NASA announced the next phase of the commercial services program, Commercial Crew Transportation Capability (CCtCap), under which NASA awarded contracts for crew transportation services to Boeing and SpaceX. Once the companies complete development and are certified by NASA, the agency will purchase two flights per year to deliver and return expedition crew. CCtCap will provide NASA and our international partners with additional vehicles to deliver crews to the ISS and expand research opportunities by enabling the crew aboard ISS to increase from six to seven. NASA also continues relationships with other U.S. companies that are developing alternative transportation systems.
Beyond commercial crew and cargo transportation services, NASA is also developing strategies to stimulate sustained economic activity in LEO. This includes leveraging the ISS; supporting a policy and regulatory environment that promotes commercialization of LEO; facilitating a robust, self-sustaining, and cost-effective supply of U.S. commercial services that accommodates public and private demands; and stimulating broad sectors of the economy discovering benefits of LEO. NASA is also leveraging industry to reinvent ground operations for a flexible, multi-user spaceport, providing launch services for both government and commercial partners. Launch complex assets once used for the space shuttle have been modernized for next-generation transportation systems.
Many of these assets also provide opportunities for the commercial space industry. For example, Boeing’s Crew Space Transportation (CST)-100 Starliner commercial spacecraft is processed in the former NASA orbiter processing facility, and SpaceX plans to use Launch Complex 39A, a former space shuttle launch pad, for commercial heavy-lift launch vehicles. These and other innovative commercial partnerships have reshaped the way NASA provides launch services through a multi-user spaceport. Over the next 10 years, commercial partners will likely increase their presence in LEO by providing more products and services to government and non-governmental customers. A mature market provides reliable, on-demand, low-cost services, freeing NASA resources for more complex missions and system development. These commercial service providers help NASA execute an ambitious deep-space human exploration strategy within the agency’s anticipated budgets.
The Proving Ground
Starting early next decade, NASA will be part of a larger international community of exploration and commercial activity in the Proving Ground—a resilient, evolvable effort to extend human presence beyond LEO. NASA identified several objectives for Proving Ground missions, which are critical steps on the journey to Mars. These objectives range from demonstrating advanced solar electric propulsion (SEP) for interplanetary cargo transportation to in-space operations and deep-space habitation. New missions and activities will become possible as NASA and its partners validate capabilities, address Proving Ground objectives, and review the specific series of near-term missions. Through these missions, we are moving toward Earth Independence and progressing together on the journey to Mars.
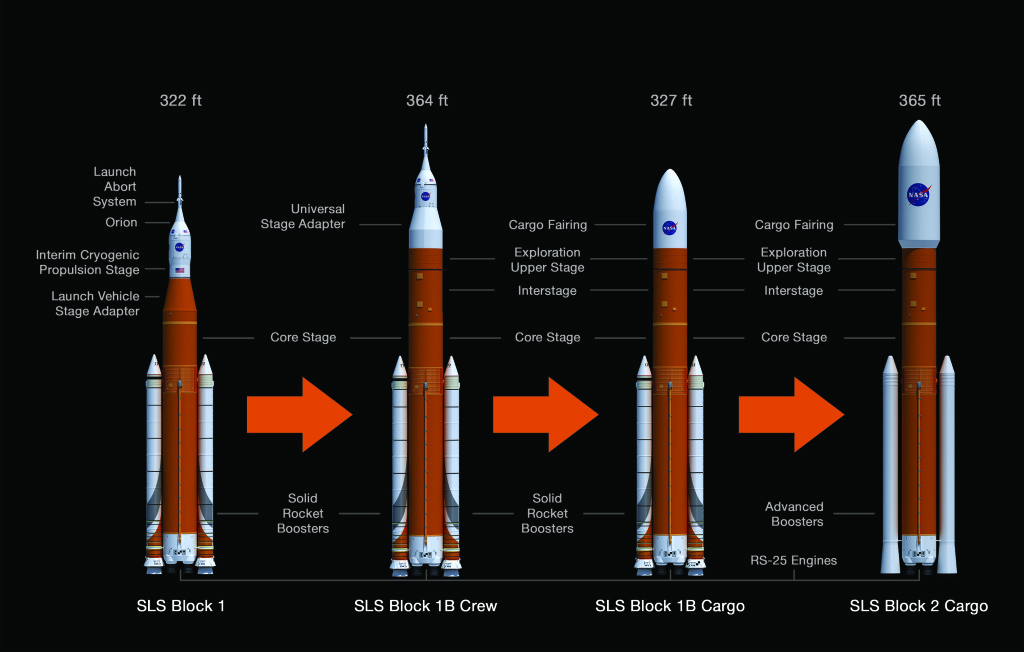
Evolution of the Nation’s Next Rocket
A Robust Transportation Infrastructure: Ground Operations, Orion, and SLS
NASA is developing a robust launch services capability, which not only supports SLS and Orion, but can also be leveraged by a multitude of new commercial launch providers. With commercial partners, the agency is modernizing Launch Complex 39B, developing a mobile launcher, upgrading control systems, and demonstrating ground processing capabilities to enable Proving Ground missions, including the launch of SLS and Orion. Orion is a launch, reentry, and in-space crew spacecraft designed to transport a crew of four to deep space. During Proving Ground missions, Orion will protect the crew during transport to cislunar space, sustain the crew for short durations while in space, and enable safe reentry.
For future missions, Orion will provide transportation between Earth and the Mars transit systems located in cislunar space. Orion’s first mission, Exploration Flight Test 1 (EFT-1), was successfully conducted in 2014, on a Delta IV Heavy launch vehicle, and generated a wealth of data to enable future human missions to deep space.
The Space Launch System is Orion’s ride to deep space. NASA is developing an evolvable design for SLS that leverages previous launch system investments.
The initial “Block 1” SLS is designed to carry Orion, as well as cargo, equipment, and science experiments to staging points in cislunar space. We are well along the path to developing the Block 1 SLS, which uses an upper stage derived from the Delta cryogenic second stage to launch 70 metric tons (mt) to orbit.
This initial version will use liquid hydrogen and liquid oxygen propulsion systems and solid rocket boosters, evolved from heritage systems. NASA plans to upgrade the boosters and develop an advanced upper stage, the Exploration Upper Stage (EUS), leading to the 105 mt Block 1B and the 130 mt Block 2 versions of the SLS. This payload capacity far exceeds the capability of current and planned commercial launch vehicles. Development of Block 1B with the EUS provides significant additional capability for Proving Ground missions, allowing NASA to send the crewed Orion spacecraft, other flight systems, and cargo to lunar orbit in a single launch. Additional developments will improve SLS performance and reduce manufacturing costs through additive manufacturing and advanced out-of-autoclave composite structures.
The initial Block 1 SLS will support the first deep-space exploration mission, Exploration Mission 1 (EM-1), anticipated in 2018. Although uncrewed, EM-1 will provide the first integrated test of SLS and Orion, including SLS’s launch performance, Orion’s heat shield, and deep-space navigation. NASA plans to develop the EUS for early exploration missions to cislunar space. The EUS addition could provide SLS with a critical new capability for crewed Orion missions by allowing secondary payloads to be co-manifested within the EUS-to-Orion launch vehicle adapter. While the exact mass and volume available for co-manifested payloads have not yet been determined, payloads about the same length, twice the width, and one-third the mass of a school bus could be launched to cislunar space with Orion.
Co-manifested payloads, potentially launched as early as Exploration Mission 2 (EM-2), could include pressurized modules that extend the deep-space capabilities of the Orion spacecraft and help develop a deep-space habitation capability. Independent co-manifested payloads, such as robotic science missions, are also possible. Orion and SLS (with the EUS) provide the core transportation capabilities that support Proving Ground missions and enable the journey to Mars. Beyond EM-2, NASA is considering a wide range of activities that not only demonstrate the ability to live and work in deep space, but also accomplish a suite of Proving Ground objectives and validate key operational capabilities required to become Earth Independent. While SLS and Orion flight rates will ultimately be determined by available funding and mission requirements, NASA is working towards flying at least one crewed mission per year.
Into the Proving Ground: Solar Electric Propulsion and the Asteroid Redirect Mission
Solar electric propulsion (SEP) uses energy from the sun to accelerate ionized propellant to very high speeds. Compared to chemical propulsion, electric propulsion provides very low levels of thrust; however, it is incredibly efficient and can provide thrust continuously for months or years, allowing more mass to be transported with far less propellant. These systems are an order of magnitude more efficient than chemical propulsion systems, with a specific impulse (Isp) from 2,000-3,000 seconds compared to 200-500 seconds. SEP systems are also very resilient and, if refueled, could provide a reusable, in-space transportation infrastructure.
Adding a SEP capability to Orion and SLS provides a robust transportation infrastructure for human missions to support the journey to Mars. High-power SEP is a key enabler for NASA’s pioneering strategy, allowing NASA to pre-position infrastructure and resources while reducing the surge of launches. This could reduce the costs estimated in previous human Mars mission studies. Pre-positioning supplies months or years ahead of crew, rather than aggregating all necessary equipment and supplies in Earth orbit, is often called a split mission. With several tons of xenon (Xe) propellant and solar arrays capable of generating 40 kilowatts (kW), an early SEP vehicle could efficiently position several tons of cargo throughout the solar system. A more advanced SEP system with additional power and propellant could deliver landers, habitats, and supplies to Mars orbit.
NASA is considering several mission architectures that evolve from a 40 kW SEP vehicle. In an approach that leverages both SEP and chemical propulsion, a Mars SEP cargo vehicle would transport chemical return stages, habitats, and landers to Mars orbit, while crew travel separately. For this approach, the crew would rendezvous with the pre-positioned assets in Mars orbit including a surface lander and, on the return trip to Earth, chemical departure stages. In an alternative approach, NASA would use a hybrid SEP vehicle, supplemented with small chemical engines for strategic high-thrust maneuvers, to reduce trip times. This vehicle would still pre-position landers, habitats, and equipment in a cargo mode; however, for crew transit, the vehicle would contain enough propellant for a round trip and would not require chemical return stages. Both approaches are being studied as the Mars transportation architecture evolves.
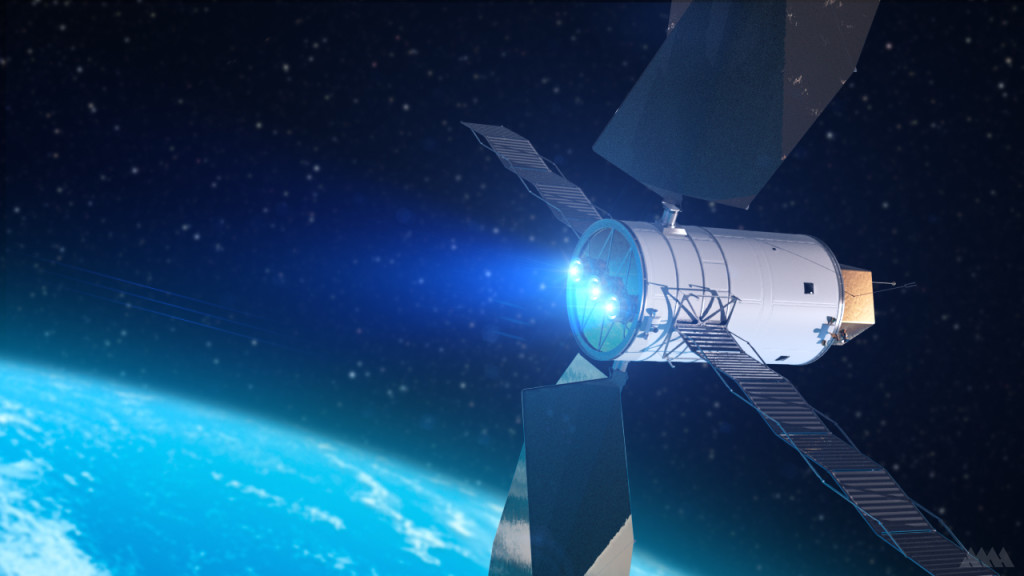
Solar Electric Propulsion (SEP) technologies is an essential part of future missions into deep space with larger payloads.
With SEP, NASA can also aggregate, refurbish, and reuse Mars transportation infrastructure in a high-energy orbit, such as lunar distant retrograde orbit (LDRO). High performance liquid oxygen and hydrogen propulsion from the SLS Exploration Upper Stage can provide the majority of departure energy, placing payloads in these high-energy orbits and allowing the SEP or SEP-hybrid thrusters to initiate the maneuver to Mars. As an added advantage, these maneuvers can be reversed, allowing NASA to capture the Mars return vehicle for refurbishment and reuse. Developing and demonstrating advanced SEP systems early during the Proving Ground missions will accelerate our progress toward sustainable pioneering. The Asteroid Redirect Mission (ARM) provides a near-term opportunity to demonstrate several capabilities important for longer-duration, deep-space missions, including flight-validated SEP transportation.
ARM consists of two challenging flight segments, working toward the common objective of human exploration of an asteroid. In the first segment, an advanced, high-power SEP robotic vehicle will travel to an asteroid, perform detailed reconnaissance of its surface, select a boulder-sized sample, capture it, and return to a stable orbit in cislunar space.
Most robotic sample return concepts are designed to return a few hundred grams of material (e.g., NASA’s OSIRIS-REx mission to the asteroid Bennu). All six Apollo landings returned a total of less than 400 kilograms.
Leveraging SEP and advanced robotics, ARM will return several tons of material. In the second segment, astronauts will travel to the captured boulder aboard SLS and Orion, marking a historic opportunity that will allow humans to venture outside of the spacecraft to touch, investigate, and experience an asteroid firsthand.
The Asteroid Redirect Mission also leaves deep-space infrastructure in cislunar space, providing an aggregation point to support the journey to Mars. Following the first ARM crewed mission, there will be on the order of several tons of material remaining in a stable orbit for at least 100 years, available for future exploration, scientific, commercial, or academic partners. During potential future missions, NASA could use the boulder to demonstrate ISRU identification, characterization, extraction, processing, and containment capabilities, and test EVA tools for exploring other low-gravity bodies, such as the Martian moons Phobos and Deimos. The Asteroid Redirect Robotic Vehicle (ARRV) also will be able to provide some support to visiting vehicles, including an S-band transponder for approach and docking, an X-band communications link, and about 40 kW of power. This initial cislunar infrastructure would be available to commercial partners who may also wish to understand and eventually mine resources from asteroids. Such commercial efforts could evolve into services to support in-space fueling of Mars propulsion systems.